Motion patterns of bacteria were studied in real time to determine how these motions related to communication within a bacterial colony, by a team of researchers.
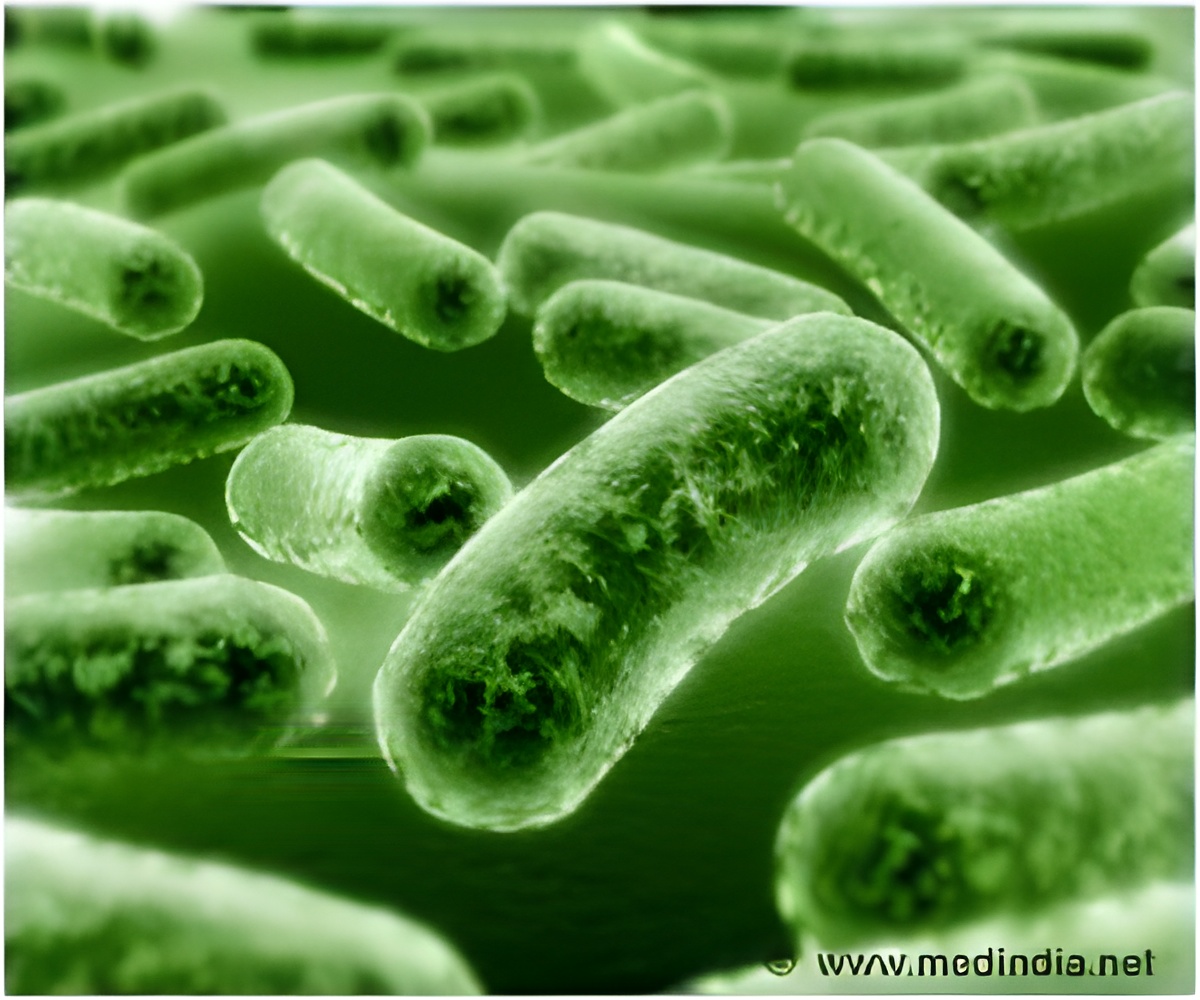
"When they die, they stop moving, so it's a good way to measure the effectiveness of an antibiotic," said Kamil Ekinci, an associate professor at Boston University. He and fellow researchers describe their work in the journal Applied Physics Letters, which is produced by AIP Publishing. "You know more or less immediately that they're dead."
The traditional method of assessing a bacteria's antibiotic susceptibility– culturing bacteria on agar plates infused with antibiotics – is quite time-consuming in comparison, and can take up to a day to produce results.
"Here in this system – down to a couple hundred of bacteria – we're able to see their responses to external stimuli such as drugs," said Utkan Demirci, an associate professor at Stanford University School of Medicine. "This also potentially applies to other types of cells, such as drug resistance in cancer."
While cantilevers have been used before to characterize cellular mechanics, Ekinci and Demirci bring a new approach to look at cellular movement and noise in natural systems, hoping to eventually develop a disposable microfluidic chip.
"It's a new direction for tool development," Demirci said. "It could allow us to address some interesting biological questions in the antibiotic resistance and evolution space."
Advertisement
"We saw that the fluctuations were focused at certain frequencies – they weren't like white noise," Ekinci said. Not quite white, but rather, something closer to pink.
Advertisement
"We think that there are several different time scales in the motion of these bacteria, and when you look at them collectively, you see 1/f-type behavior," Ekinci said.
In addition to the long-term goal of creating smaller, portable sensors, future work includes identifying the precise structural sources of vibrations, in order to develop a quantitative physical model of the noise and better understand the bacterial communication pathways.
"I want to make this more quantitative and determine the sources of these noises," Ekinci said. "I think this could be a useful tool for doing some fundamental studies."
Source-Eurekalert